By Sarah Garlick, Alexandra R. Contosta, Nora J. Casson, Sarah J. Nelson, Matthew P. Ayres, Elizabeth A. Burakowski, John Campbell, Irena Creed, Catherine Eimers, Celia Evans, Ivan Fernandez, Colin Fuss, Thomas Huntington, Kaizad Patel, Rebecca Sanders-DeMott, Kyongo Son, Pamela Templer, Casey Thornbrugh
September 30, 2020
Winter Conditions are Changing Across the Northern Forest
Winter climate change may fundamentally reshape the ecological and social fabric of the northern forest.
Winter is warming faster than any other season in eastern North America. A group of scientists from the U.S. and Canada examined one hundred years of winter temperature and precipitation data from weather stations across the northern forest region (Figure 1). The results show a clear picture of declining snowpack and fewer days with cold temperatures over the last century. This loss of cold and snow, in a region historically adapted to cold, snowy winters, has profound implications for water, wildlife, forests, and people. “Whether precipitation falls as snow or rain makes a huge difference — whether you’re a skier, a snowshoe hare, or a forest stream,” said Dr. Alexandra Contosta, the lead scientist of our synthesis study. Winter climate change may fundamentally reshape the ecological and social fabric of the northern forest region.
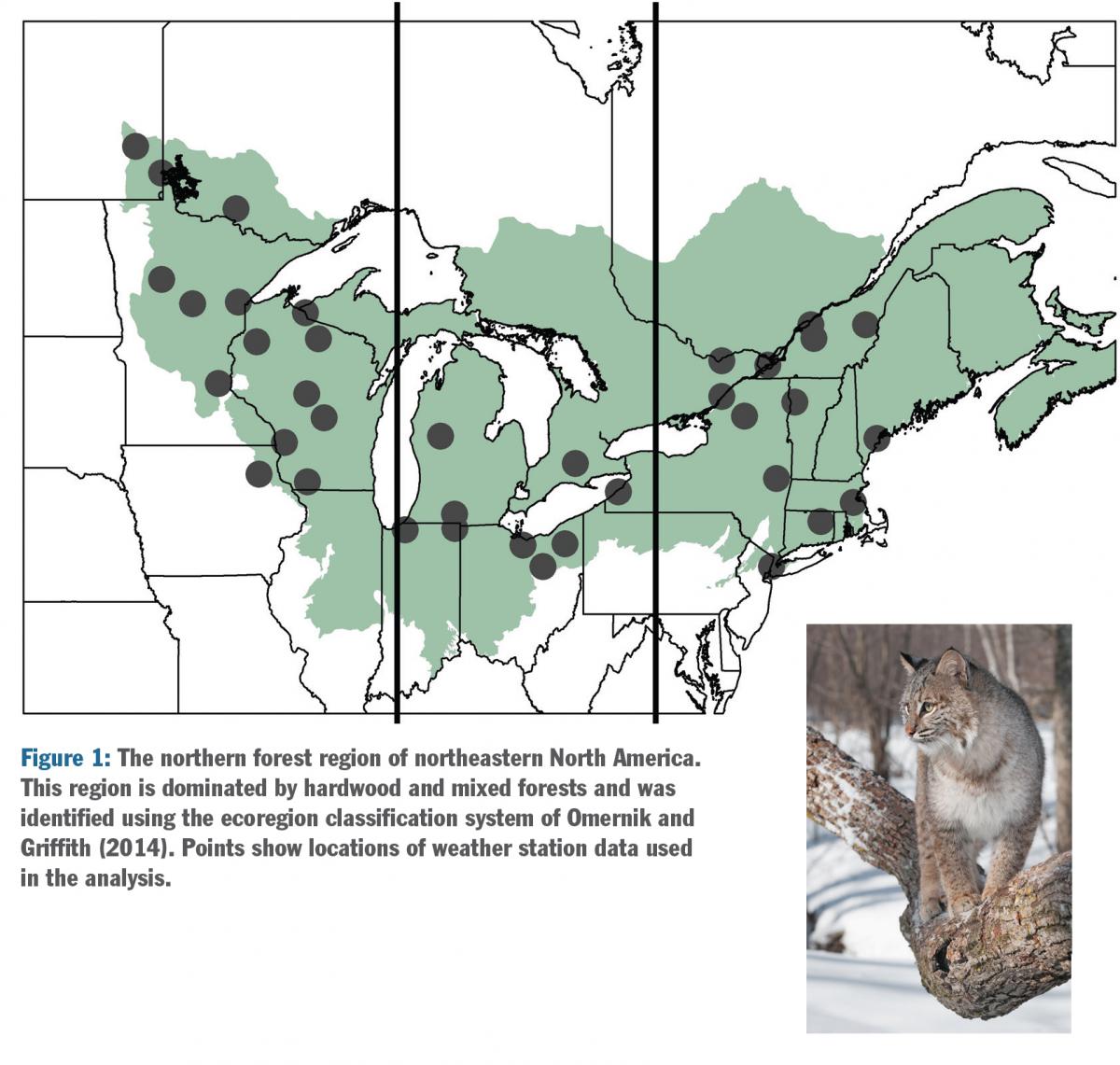
We calculated specific indicators of how winter conditions have changed across the northern forest over the past one hundred years: climate metrics such as the number of frost days, the number of snow-covered days, and the number of days of extreme cold. We invite you to explore these indicators below, along with general trends in winter climate data for the region.
We developed these indicators in dialogue with community leaders and stakeholders from various networks, including outdoor recreation and tourism, land management and conservation, and the forest products industry. We heard about the winter conditions that matter most to ski areas, logging operations, and land management planning, and we calculated the trends in those conditions over the past century.
Download a PDF version of this report.
This report summarizes research from the following sources:
Contosta et al. (2019) Northern forest winters have lost cold, snowy conditions that are important for ecosystems and human communities. Ecological Applications, 29(7)
Contosta et al. (2019) Defining frigid winter illuminates its loss across seasonally snow-covered areas of eastern North America. Environmental Research Letters
The changes shared in this report are not forecasts for the future; they are what we are seeing right now, on the ground, across the northern forest. We are also seeing people taking action. Snowmobilers are building trails through the woods that allow them to ride on thinner snowpack. Timber harvesting operations are shifting into the dry parts of summer instead of relying only on winter. Community members are inspiring collective action in their towns to lower greenhouse gas emissions. We hope this synthesis report provides evidence to guide these actions and others as we all confront our changing winters.
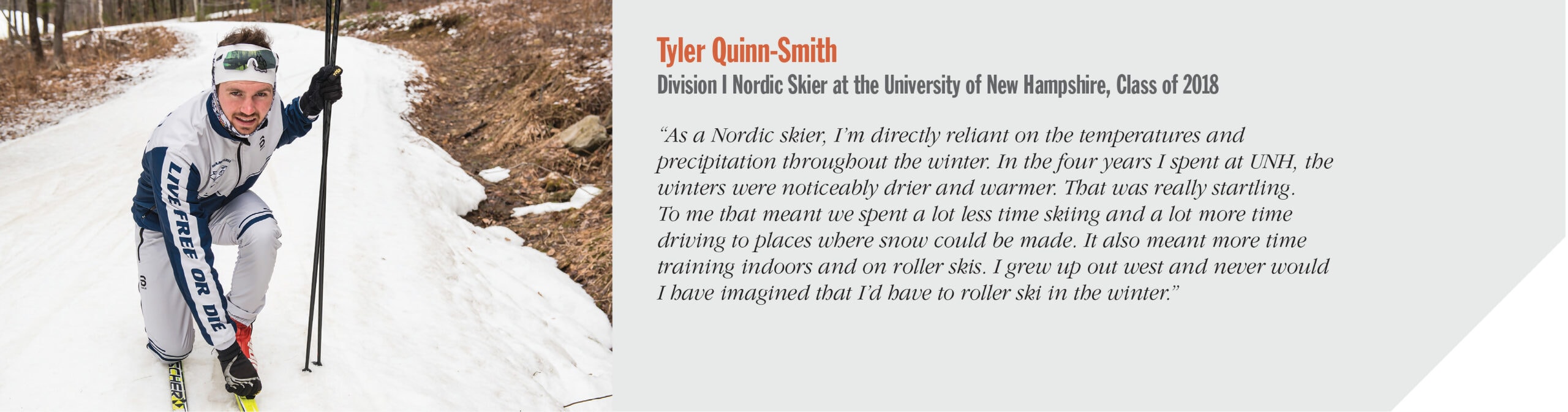
Key Findings
1) We are losing the cold.
We found overall declining trends in the indicators of “coldness.” For example, there are now fewer ice days (when daytime temperatures never go above freezing), frost days (when nighttime temperatures dip below freezing and there is a frost), and extreme cold days (when temperatures go below 0º F) than in the past 100 years. The loss of frost days over the past century was most apparent, with decreases of 18 days in the eastern region of the northern forest, 15 days in the central region, and 11 days in the western region.
2) We are losing the snow.
Winters in the eastern areas of the northern forest have lost 21 days of snow cover over the past century. Winters in the western areas have lost 17 days of snow cover over the past century. In both of these regions, we found increases in the number of “mud days” in winter: days when the ground is bare and daytime temperatures go above freezing. Weather stations in the central northern forest show mixed trends, with some sites near the Great Lakes gaining snow-covered days. The increase in snow-covered days near the Great Lakes likely reflects an increase in lake-effect snowfalls, which are driven by a combination of warming water, reduced ice cover, and increased evaporation.
3) Winters are getting shorter.
The cold period of the year has shortened by about three weeks across the northern forest. During the 1910s, the sustained cold period lasted about 146 days (range of 85–190 days across individual sites). During the 2010s, the sustained cold period was down to about 126 days (range of 9–194 days across individual sites). This shortening of the length of the cold, snowy period of the year is largely due to earlier onset of spring. Also, during this cold period, cold and snowy conditions have become more intermittent. For instance, in the central region in the 1910s, about three quarters of the sites had snow cover on January 1st, but by the 2010s only about half the sites had snow cover on January 1st.
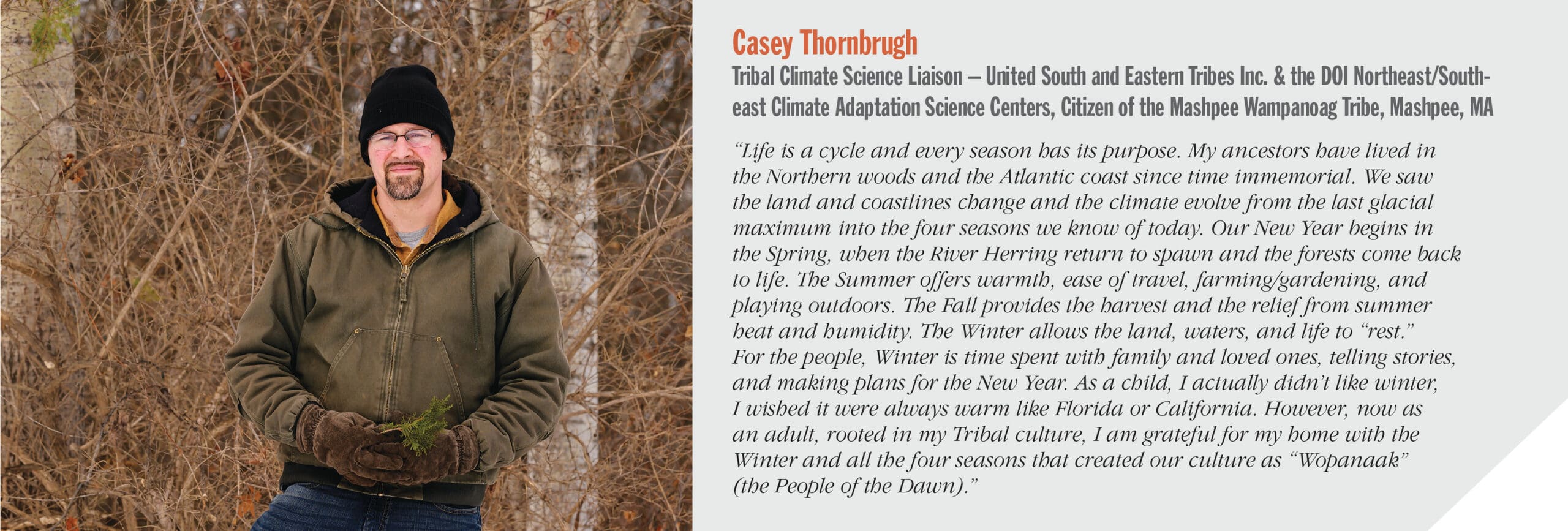
Indicators of Change
Twelve climate metrics illustrate how winter conditions have changed over the past 100 years. Geographic subregions correspond with the east, central, and west subregions of the maps shown above in Figure 2.
1. Length of Winter
Change over the past 100 years in the length of the sustained cold period of the year:
- 21 fewer days: northeastern U.S. and Atlantic Canada
- 24 fewer days: Great Lakes region
- 14 fewer days: upper midwest U.S. and Canadian southern prairies
2. Frost days
Change over the past 100 years in the number of days when minimum daily temperatures dip below freezing.
- 18 fewer days: northeastern U.S. and Atlantic Canada
- 15 fewer days: Great Lakes region
- 11 fewer days: upper midwest U.S. and Canadian southern prairies
3. Ice days
Change over the past 100 years in the number of days when maximum daily temperatures remain below freezing.
- 14 fewer days: northeastern U.S. and Atlantic Canada
- 13 more days: Great Lakes region
- 13 fewer days: upper midwest U.S. and Canadian southern prairies
4. Thaw days
Change over the past 100 years in the number of days when maximum daily temperatures rise above freezing.
- 18 more days: northeastern U.S. and Atlantic Canada
- 12 fewer days: Great Lakes region
- 12 more days: upper midwest U.S. and Canadian southern prairies
5. Extreme cold days
Change over the past 100 years in the number of days when minimum daily temperatures reach below 0ºF (-18ºC). These are conditions that kill invasive southern pine beetles.
- 15 fewer days: northeastern U.S. and Atlantic Canada
- 6 more days: Great Lakes region
- 14 fewer days: upper midwest U.S. and Canadian southern prairies
6. Hemlock woolly adelgid kill days
Change over the past 100 years in the number of days cold enough to kill the invasive hemlock woolly adelgid (min daily temp below -22ºF).
- no change: northeastern U.S. and Atlantic Canada
- no change: Great Lakes region
- 5 fewer days: upper midwest U.S. and Canadian southern prairies
7. Snowmaking days before Christmas
Change over the past 100 years in the number of days before December 25 with temperatures cold enough to make snow (minimum daily temperatures below 23ºF). This is a key period for ski area operations.
- 16 fewer days: northeastern U.S. and Atlantic Canada
- 14 more days: Great Lakes region
- 15 fewer days: upper midwest U.S. and Canadian southern prairies
8. Invasive mosquito kill days
Change over the past 100 years in the number of days cold enough to kill the invasive Asian tiger mosquito, a carrier of human diseases (minimum daily temperatures below 23ºF).
- 16 fewer days: northeastern U.S. and Atlantic Canada
- 1 more day: Great Lakes region
- 9 fewer days: upper midwest U.S. and Canadian southern prairies
9. Snow-covered days
Change over the past 100 years in the number of days with snow depth greater than 0 mm.
- 21 fewer days: northeastern U.S. and Atlantic Canada
- no change: Great Lakes region
- 17 fewer days: upper midwest U.S. and Canadian southern prairies
10. Rain-on-snow days
Change over the past 100 years in the number of days when precipitation falls as rain when snow depth is greater than 0 mm.
- 5 fewer days: northeastern U.S. and Atlantic Canada
- no change: Great Lakes region
- 1 more day: upper midwest U.S. and Canadian southern prairies
11. Frozen ground days
Change over the past 100 years in the number of days with bare ground plus ice (maximum daily temperature below freezing and snow depth = 0 mm).
- no change: northeastern U.S. and Atlantic Canada
- 4 fewer days: Great Lakes region
- no change: upper midwest U.S. and Canadian southern prairies
12. Mud days
Change over the past 100 years in the number of days with bare ground plus thaw (maximum daily temperature above freezing and snow depth = 0 mm).
- 20 more days: northeastern U.S. and Atlantic Canada
- no change: Great Lakes Region
- 15 more days: upper midwest U.S. Canadian southern prairies
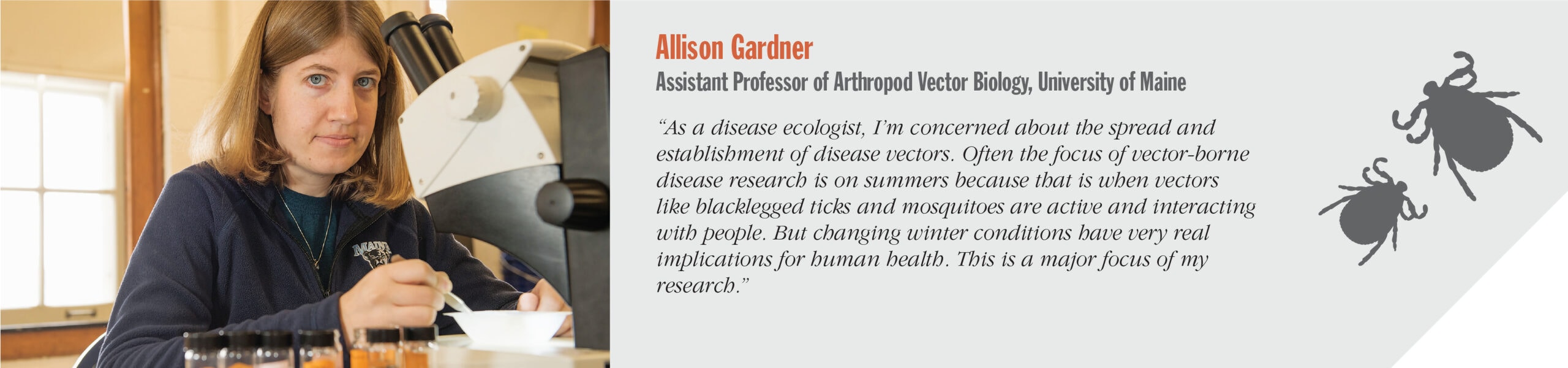
Why It Matters: Impacts of Changing Winters
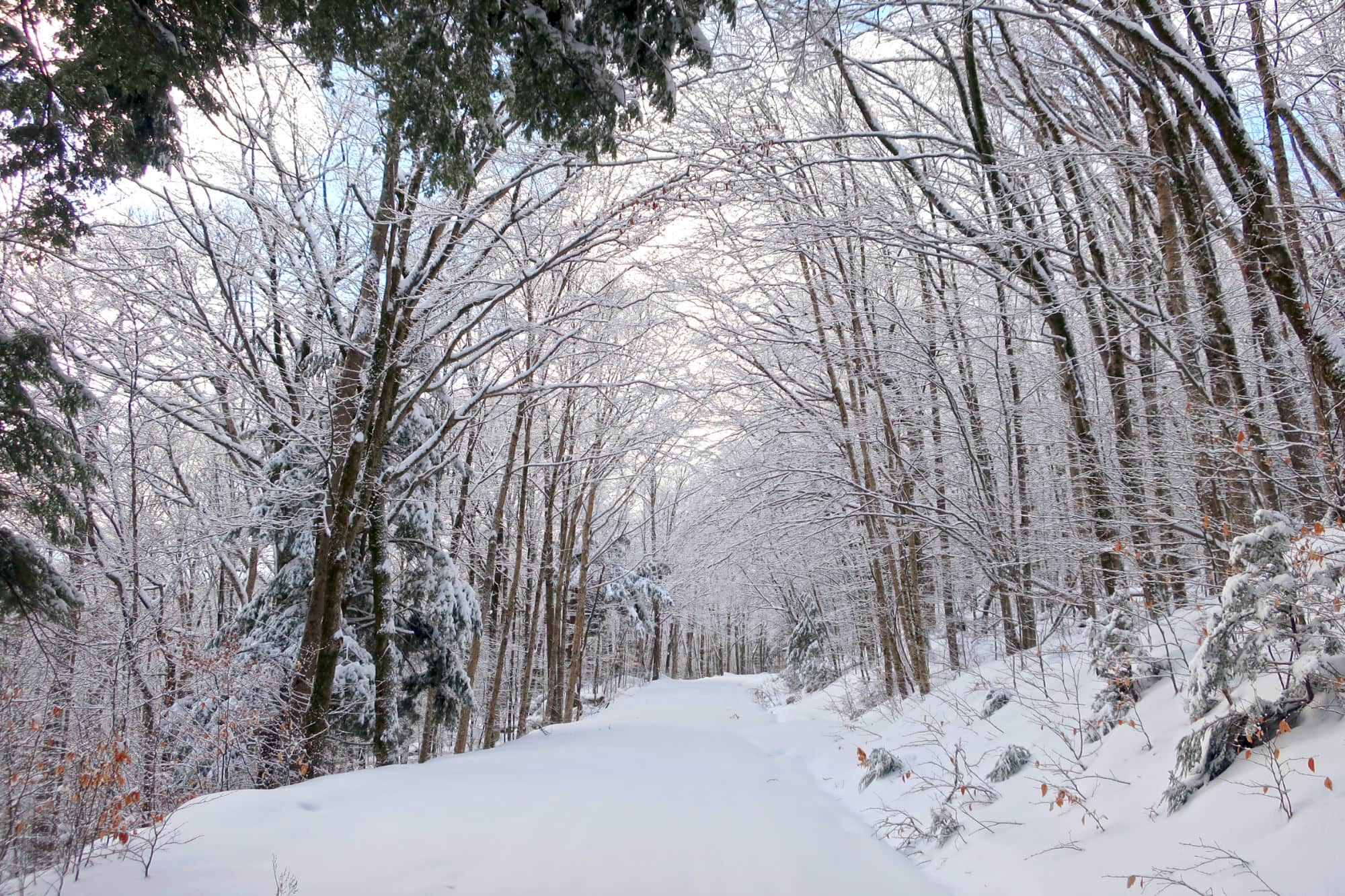
Forests
Changing winter conditions can affect forest soils, nutrient availability, tree health, and vulnerability to invasive forest insects and diseases.
Paradoxically, loss of the insulating snowpack can lead to frozen soils, which can damage tree roots and cause decreased growth the following season.
Frozen soils can also change the ability of trees to retain nutrients and carbon.
Increases in mud days and winter rainfall can result in wet soils. If refrozen, this can lead to concrete frost formation, altering soil carbon and nitrogen availability, reducing permeability, and increasing surface water runoff.
The ranges of invasive and damaging forest pests like the southern pine beetle and the hemlock woolly adelgid are constrained by cold winter temperatures that kill off many of the pests.
For the forest products industry, the loss of snowpack and an increase in mud days mean fewer days when conditions are suitable for winter logging. Without a protective snowpack, the ground is vulnerable to damage from logging equipment.
Water
Changing winter conditions can affect municipal water supplies, the quality and safety of stream water, the risk of winter flooding events, and the duration and thickness of lake ice.
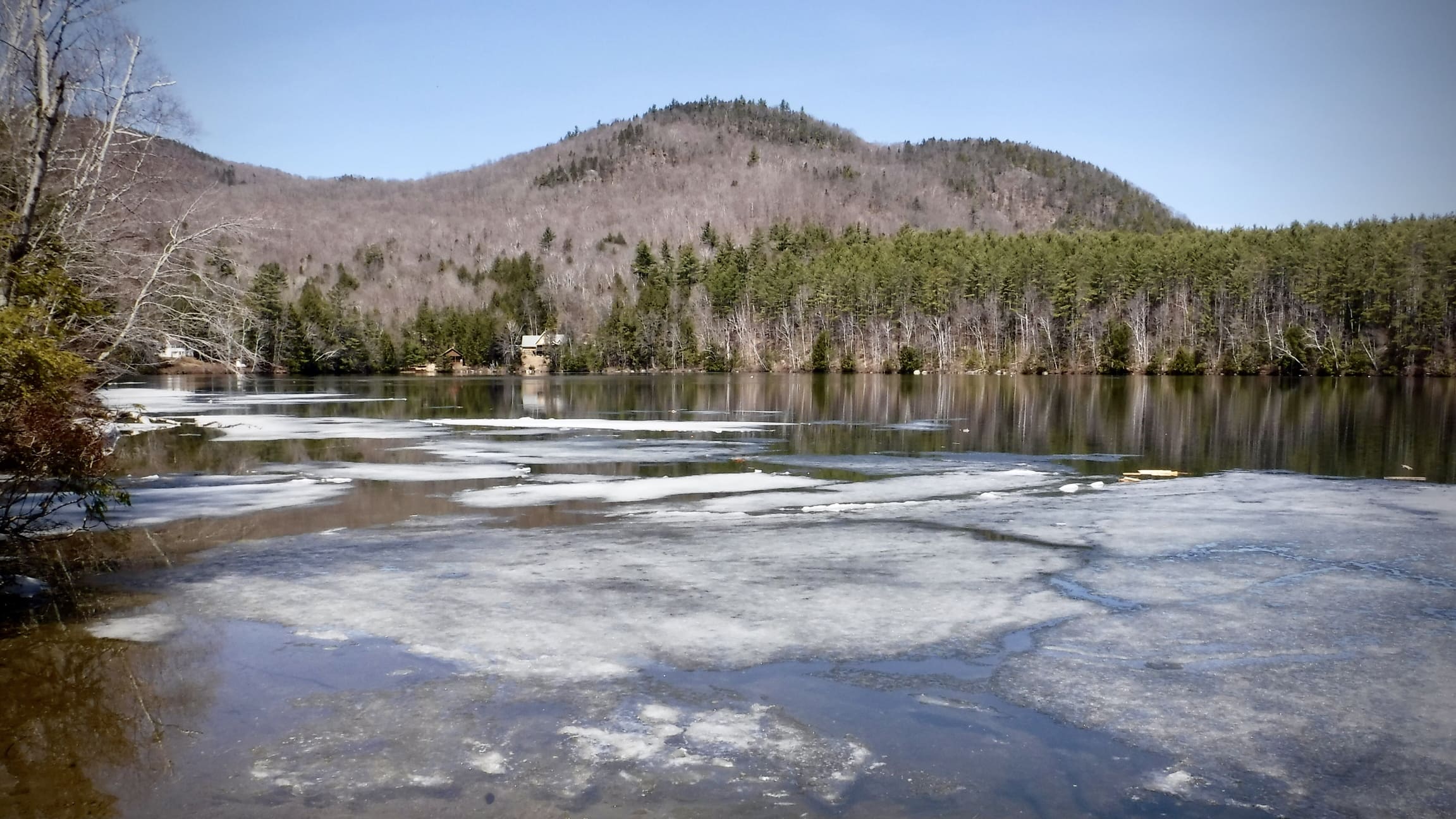
Warmer winter temperatures result in more frequent melting events and earlier spring snow melt.
More frequent winter rains and increased winter runoff can increase nitrate export in stream water and cause potential acid pulses.
More frequent winter rains can cause ice jams and damaging floods.
Across North America and the globe, lake ice-out dates are occurring earlier in the year.
The loss of lake ice negatively impacts lake ecology and can disrupt winter recreation, including snowmobiling, ice fishing, and pond hockey.
Wildlife
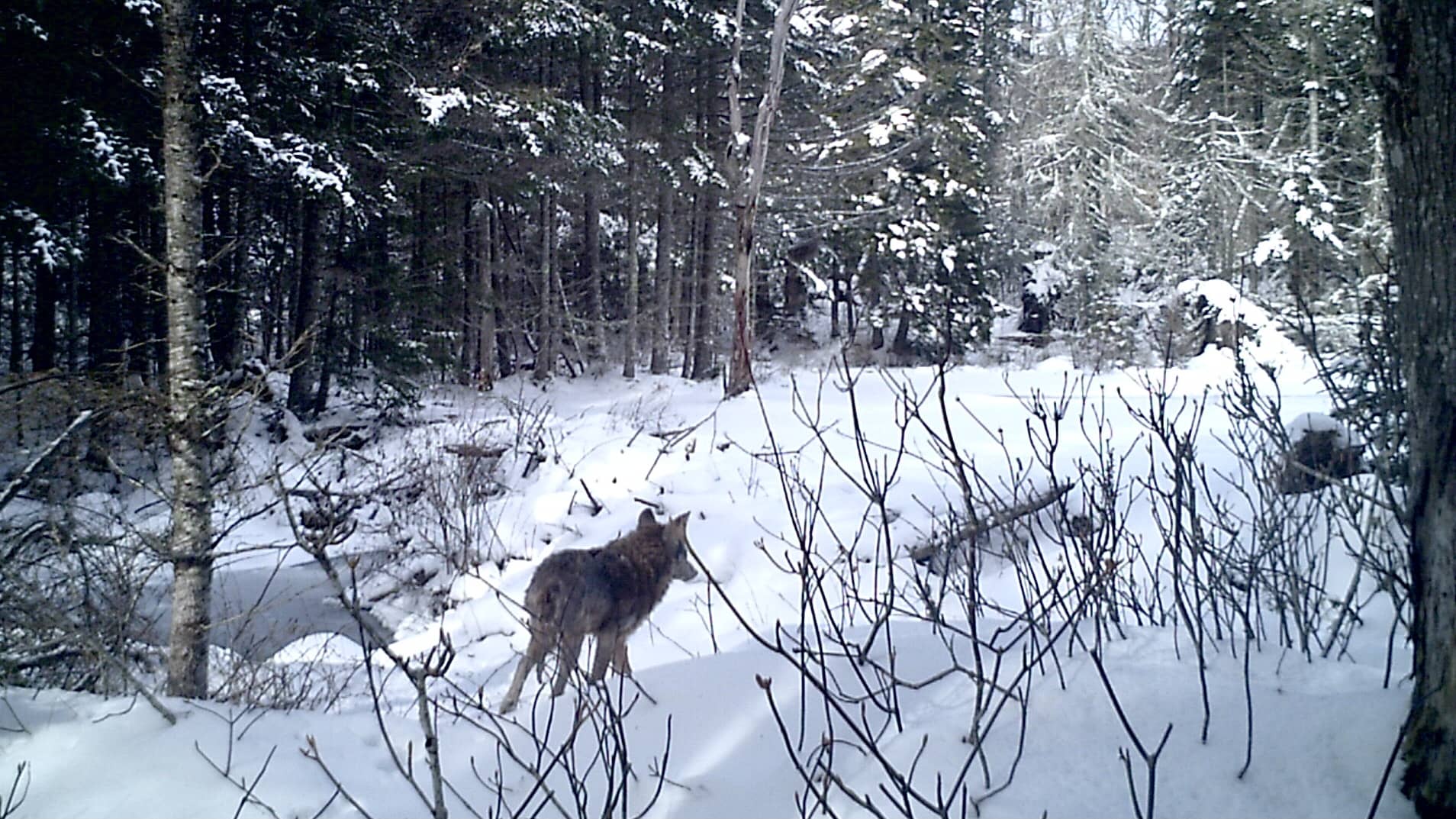
Changing winter conditions can affect wildlife habitat, wildlife populations, predator–prey relationships, and vulnerability of wildlife to parasites and diseases.
Changes in the depth, physical characteristics, and timing of the snowpack can negatively affect under-snow habitat and reduce small mammal abundance. This can lead to enhanced competition among predators, for example allowing red fox to outcompete Arctic fox for food resources.
Reduced snow depth or formation of ice crusts in warmer and wetter winters could impede the ability of ruffed grouse to snow roost, potentially leading to population decline.
Changes in the length of the snow-covered season can create camouflage mismatch for snowshoe hare, which is important prey for Canada lynx and other predators including coyotes, goshawks, and great horned owls.
Moose have been affected by parasites such as winter tick that can flourish during warmer winters.
White-tailed deer survival, on the other hand, can increase with warmer winters.
For freshwater aquatic organisms, decreases in coldness or snow cover may alter the availability of spring insect prey to fish such as brook trout and Atlantic salmon.
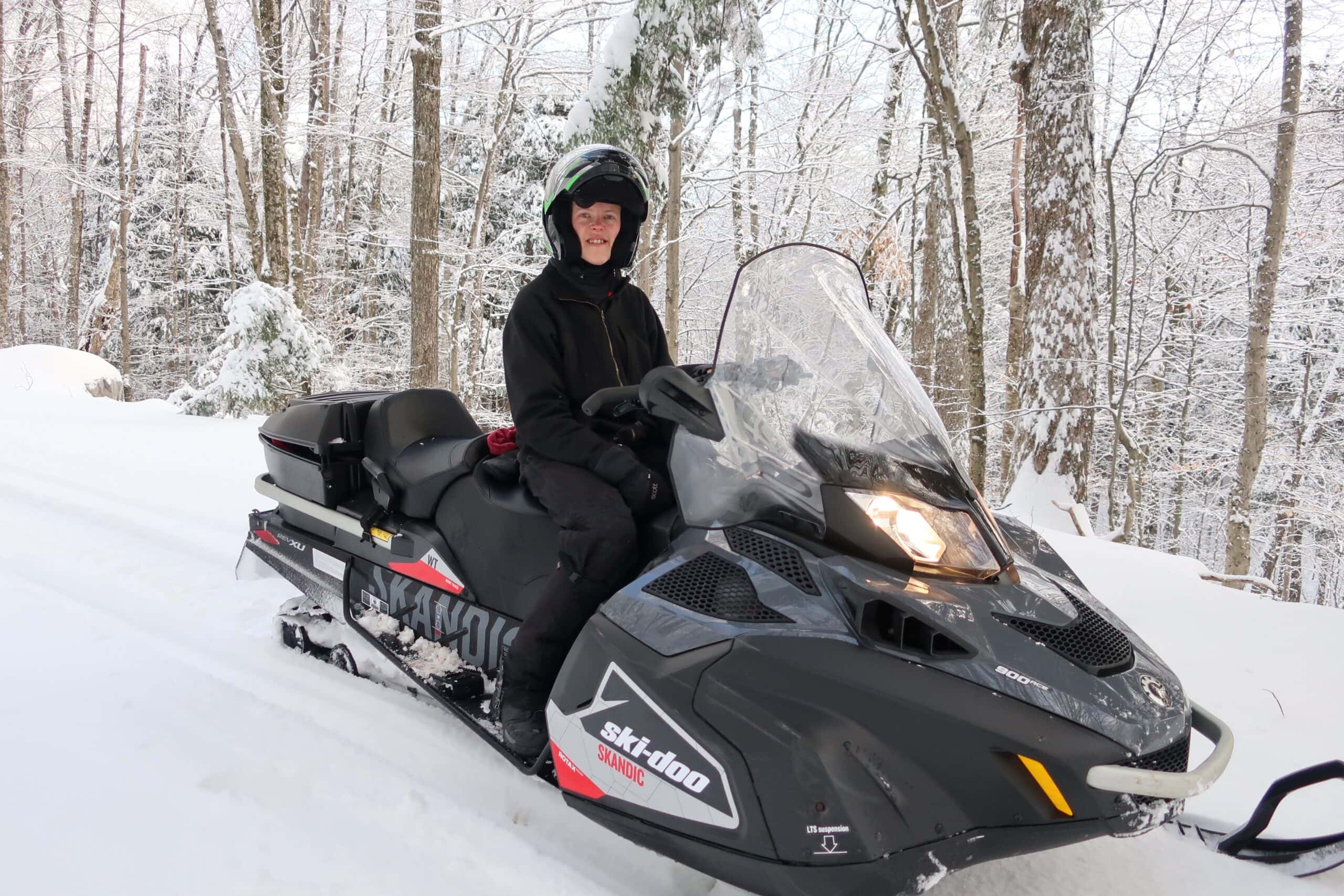
People
Changing winter conditions have direct impacts on people. Human health, cultural practices and traditions, winter recreation and tourism, and winter logging operations and the forest products industry are all affected by loss of cold and snow.
Milder winter temperatures play a role in the geographic distribution of the blacklegged tick that carries the bacteria that causes Lyme disease.
Winter temperatures also control the geographic distribution of the invasive Asian tiger mosquito, which can carry dengue and chikungunya fevers as well as eastern equine encephalitis.
The northern forest region is the original homeland of many Indigenous peoples whose cultural practices and traditional knowledge are interconnected with specific places, ecological processes, and species. The ecological impacts of climate change are thus compounded within these communities, including impacts on physical and mental health. Further exacerbating these effects are stressors related to colonialism, systemic racism, and forced relocation.
Winter recreation is a multi-billion-dollar industry in the U.S. and Canada. Many ski area operators are adapting to changing winter conditions with snowmaking. The technology for snowmaking has, thus far, continued to improve, allowing snowmaking outputs to increase despite the decreases in suitable snowmaking days.
However, not all winter recreation pursuits can benefit from snowmaking, like snowmobiling. This means a significant potential loss of winter tourism revenue to many towns in the northern forest.
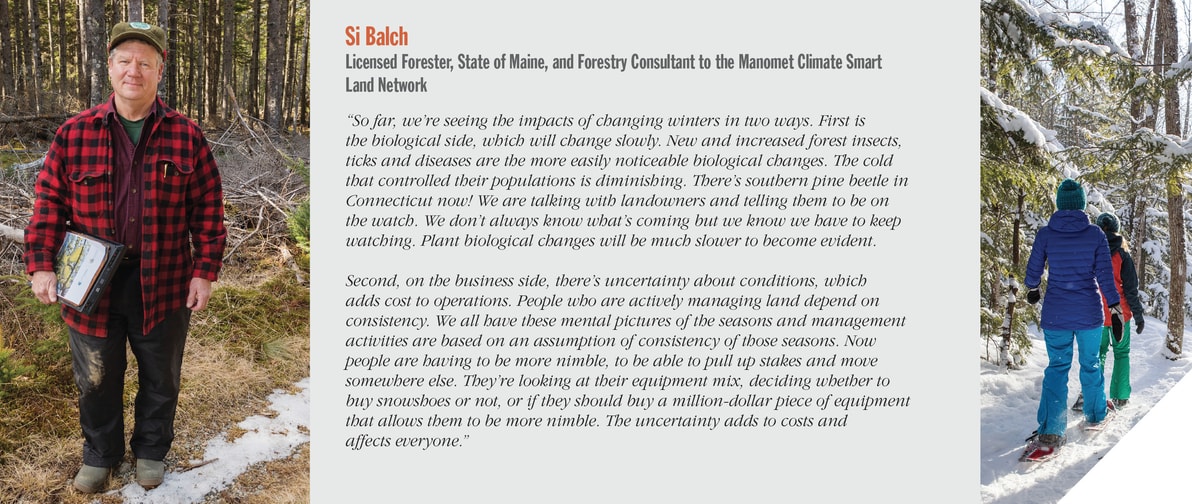
Acknowledgments and References
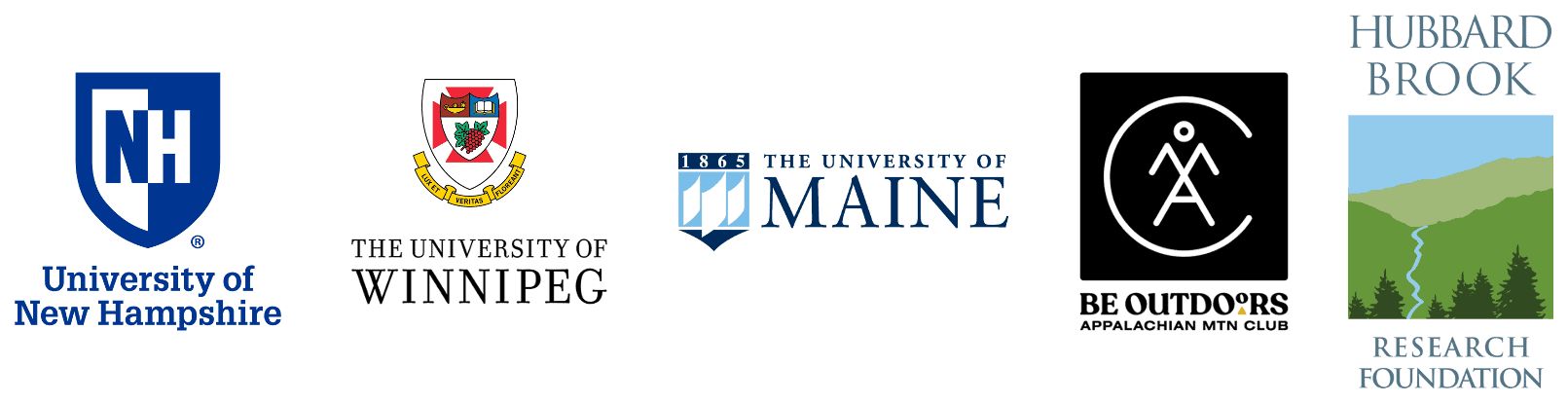
The mission of the Hubbard Brook Research Foundation is to promote the understanding and stewardship of ecosystems through scientific research, long-term monitoring, policy outreach, and education. This publication is a contribution of a collaborative project led by the University of New Hampshire, Hubbard Brook Research Foundation, University of Winnipeg, University of Maine, and Appalachian Mountain Club. This project was funded by the Northeastern States Research Cooperative (NSRC), Canaday Family Charitable Trust, Lintilhac Foundation, and Davis Conservation Foundation. This report was also supported by the National Science Foundation’s Long Term Ecological Research program at the Hubbard Brook Experimental Forest (NSF DEB #1637685) and the National Science Foundation’s Advancing Informal STEM Learning program (NSF DRL #1713204). The Hubbard Brook Experimental Forest is operated and maintained by the USDA Forest Service, Northern Research Station, Newton Square, PA.
Authors
Sarah Garlick, Hubbard Brook Research Foundation; Alexandra R. Contosta, University of New Hampshire; Nora J. Casson, University of Winnipeg; Sarah J. Nelson, Appalachian Mountain Club; University of Maine; Matthew P. Ayres, Dartmouth College; Elizabeth A. Burakowski, University of New Hampshire; John Campbell, USDA Forest Service Northern Research Station; Irena Creed, University of Saskatchewan; Catherine Eimers, Trent University; Celia Evans, Paul Smith’s College; Ivan Fernandez, University of Maine; Colin Fuss, Cary Institute of Ecosystem Studies; Center for Earth and Environmental Science, SUNY-Plattsburgh; Thomas Huntington, United States Geological Survey; Kaizad Patel, University of Maine; Rebecca Sanders-DeMott, University of New Hampshire; Kyongo Son, Research Foundation of the City University of New York; Pamela Templer, Boston University; Casey Thornbrugh, United South and Eastern Tribes, Inc., and DOI Northeast & Southeast Climate Adaptation Science Centers
Art and Design
Title video by Joe Klementovich. Portraits by Joe Klementovich. Graphic design by Linda Mirable, Ravenmark. Editing by Clara Chaisson and Anthea Lavallee.
References
Brownstein, J. S., T. R. Holford, and D. Fish. 2003. A climate- based model predicts the spatial distribution of the Lyme disease vector Ixodes scapularis in the United States. Environmental Health Perspectives 111:1152–1157.
Casson, N. J., M. C. Eimers, and S. A. Watmough. 2012. Impact of winter warming on the timing of nutrient export from forested catchments. Hydrological Processes 26:2546–2554.
Crossman, J., et al. 2016. Regional meteorological drivers and long term trends of winter-spring nitrate dynamics across watersheds in northeastern North America. Biogeochemistry 130:247–265.
Dodds, K. J., C. F. Aoki, A. Arango-Velez, J. Cancelliere, A. W. D’Amato, M. F. DiGirolomo, and R. J. Rabaglia. 2018. Expansion of southern pine beetle into northeastern forests: Management and impact of a primary bark beetle in a new region. Journal of Forestry 116:178–191.
Dudley, R. W., G. A. Hodgkins, M. R. McHale, M. J. Kolian, and B. Renard. 2017. Trends in snowmeltrelated streamflow timing in the conterminous United States. Journal of Hydrology 547:208–221.
Dunfey-Ball, K. R. 2017. Moose density, habitat, and winter tick epizootics in a changing climate. Dissertation. University of New Hampshire, Durham, New Hampshire, USA.
Eimers, M. C., J. M. Buttle, and S. A. Watmough. 2007. The contribution of rain-on-snow events to annual NO3-N export from a forested catchment in south-central Ontario, Canada. Applied Geochemistry 22:1105–1110.
Evans, A. M., M. Lynch, F. Clark, G. M. Mickel, K. Chapman, M. Hayne, E. R. Tiller, and A. Mahaffey. 2016. Wisconsin forest practices and harvesting constraints assessment. Forest Stewards Guild, Madison, Wisconsin, USA.
Feierabend, D., and K. Kielland. 2015. Seasonal effects of habitat on sources and rates of snowshoe hare predation in Alaskan boreal forests. PLoS ONE 10:e0143543.
Fitzpatrick, M. C., E. L. Preisser, A. Porter, J. Elkinton, and A. M. Ellison. 2012. Modeling range dynamics in heterogeneous landscapes: Invasion of the hemlock woolly adelgid in eastern North America. Ecological Applications 22:472–486.
Hodgkins, G. A., R. W. Dudley, and T. G. Huntington. 2003. Changes in the timing of high river flows in New England over the 20th century. Journal of Hydrology 278:244–252.
Kurian, L. M., L. K. Lautz, and M. J. Mitchell. 2013. Winter hydrology and NO3-concentrations in a forested watershed: a detailed field study in the Adirondack Mountains of New York. Journal of the American Water Resources Association 49:264–283.
Lavigne, G. R. 1999. White-tailed deer assessment and strategic plan 1997. Maine Department of Inland Fisheries and Wildlife, Augusta, Maine, USA.
Levi, T., F. Keesing, K. Oggenfuss, and R. S. Ostfeld. 2015. Accelerated phenology of blacklegged ticks under climate warming. Philosophical Transactions of the Royal Society B 370:1–8.
McBoyle, G., D. Scott, and B. Jones. 2007. Climate change and the future of snowmobiling in nonmountainous regions of Canada. Managing Leisure 12:237–250.
Norton-Smith, K., K. Lynn, K. Chief, K. Cozzetto, J. Donatuto, M. Hiza Redsteer, L. E. Kruger, J. Maldonado, C. Viles, and K. P. Whyte. 2016. Climate change and indigenous peoples: a synthesis of current impacts and experiences. U.S. Department of Agriculture, Forest Service, Pacific Northwest Research Station, Portland, Oregon, USA.
Ogden, N. H., R. Milka, C. Caminade, and P. Gachon. 2014. Recent and projected future climatic suitability of North America for the Asian tiger mosquito Aedes albopictus. Parasites and Vectors 7:532.
Omernik, J. M., and G. E. Griffith. 2014. Ecoregions of the Conterminous United States: Evolution of a Hierarchical Spatial Framework. Environmental Management 54:1249–1266.
Patel, K. F., C. Tatariw, J. D. MacRae, T. Ohno, S. J. Nelson, and I. J. Fernandez. 2018. Soil carbon and nitrogen responses to snow removal and concrete frost in a northern coniferous forest. Canadian Journal of Soil Science 98:1–12.
Penczykowski, R. M., B. M. Connolly, and B. T. Barton. 2017. Winter is changing: trophic interactions under altered snow regimes. Food Webs 13:80–91.
Reinmann, A. B., and P. H. Templer. 2016. Reduced winter snowpack and greater soil frost reduce live root biomass and stimulate radial growth and stem respiration of red maple (Acer rubrum) trees in a mixed-hardwood forest. Ecosystems 19:129–141.
Sanders-DeMott, R., P. O. Sorensen, A. B. Reinmann, and P. H. Templer. 2018. Growing season warming and winter freeze–thaw cycles reduce root nitrogen uptake capacity and increase soil solution nitrogen in a northern forest ecosystem. Biogeochemistry 137:337–349.
Scott, D., and G. McBoyle. 2007. Climate change adaptation in the ski industry. Mitigation and Adaptation Strategies for Global Change 12:1411–1431.
Scott, D., J. Dawson, and B. Jones. 2008. Climate change vulnerability of the US Northeast winter recreation-tourism sector. Mitigation and Adaptation Strategies for Global Change 13:577–596.
Shanley, J. B., and A. Chalmers. 1999. The effect of frozen soil on snowmelt runoff at Sleepers River, Vermont. Hydrological Processes 13:1843–1857.
Templer, P. H., et al. 2017. Climate Change Across Seasons Experiment (CCASE): a new method for simulating future climate in seasonally snow-covered ecosystems. PLoS ONE 12:e0171928.
Thompson III, F. R., and E. K. Fryzel. 1988. Ruffed grouse winter roost site preference and influence on energy demands. Journal of Wildlife Management 52:454–460.
Whyte, K. 2017. Indigenous climate change studies: indigenizing futures, decolonizing the anthropocene. English Language Notes 55:153–162.
Zimmerman, G. S., R. R. Horton, D. R. Dessecker, R. J. Gutierrez, and A. R. J. Gutierrez. 2008. New insight to old hypotheses: ruffed grouse population cycles. The Wilson Journal of Ornithology 120:239–247.
Zimova, M., L. S. Mills, and J. J. Nowak. 2016. High fitness costs of climate change-induced camouflage mismatch. Ecology Letters 19:299–307.